Drug Transport via Nanocarrier for Liver Cancer Treatment
DOI:
https://doi.org/10.58567/ci01010001Keywords:
Nanocarrier (NC); Theragnostic; Liver cancerAbstract
The requirement of having multiple nanocarriers (NCs) and active agents for improved therapy, imaging, and controlled release of medications efficiently in one platform has made the creation of therapeutics and theragnostic nanodrug delivery systems a difficult task for present researchers. Multiple drug resistance (MDR), a high clearance rate, severe side effects, undesirable drug distribution to the specific site of liver cancer, and a low concentration of medication that reaches liver cancer cells are just a few of the drawbacks of traditional liver cancer chemotherapy. As a result, new techniques and NCs must be developed to transport the medication molecules targeted to the malignant hepatocytes in an acceptable number and duration inside the therapeutic window. Because of the great efficacy of drug loading or drug encapsulation efficiency, high cellular uptake, high drug release, and minimal adverse effects, therapeutics and theragnostic systems have benefits over conventional chemotherapy. These NCs have a high drug accumulation rate in tumours while causing minimal toxicity in healthy tissues. This study focuses on current research on NC-based therapies and theragnostic drug delivery systems, omitting nanotechnology's negative consequences in the field of drug delivery systems. Clinical advancements of theragnostic NCs for liver cancer, on the other hand, are not covered in this article. Only the most current breakthroughs in NC-based drug delivery systems for liver cancer therapy and diagnosis are discussed in this study. This review will not go over the detrimental effects of individual NCs in the medication delivery system.
References
Jemal A, Bray F, Center M. Global cancer statistics. CA Cancer J. Clin. 2011; 61(2): 69–90. doi:10.3322/caac.20107
Sia D, Villanueva A, Friedman SL, et al. Liver cancer cell of origin, molecular class, and effects on patient prognosis. Gastroenterology. 2017; 152: 745–761. doi:10.1053/j.gastro.2016.11.048
Azzariti A, Iacobazzi RM, Fanizza E, et al. Sorafenib delivery nanoplatform based on superparamagnetic iron oxide nanoparticles magnetically targets hepatocellular carcinoma. Nano. Res. 2017; 10(7): 2431–2448. doi:10.1007/s12274-017-1444-3
Chabner BA, Roberts TG. Chemotherapy and the war on cancer. Nat. Rev. Cancer. 2005; 5(1): 65. doi:10.1038/nrc1529
Kiparissides C, Kammona O. Nanoscale carriers for targeted delivery of drugs and therapeutic biomolecules. Can. J. Chem. Eng. 2013; 91(4): 638–651. doi:10.1002/cjce.v91.4
Zhang N, Yu R, Cheng XY, et al. Visual targeted therapy of hepatic cancer using homing peptide modified calcium phosphate nanoparticles loading doxorubicin guided by T1 weighted MRI. Nanomedicine 2018. doi:10.1016/j.nano.2018.06.014
Mishra N, Yadav NP, Rai VK, et al. Efficient hepatic delivery of drugs: Novel strategies and their significance. Biomed. Res. Int. 2013; 2013. doi:10.1155/2013/382184
Lata S, Sharma G, Joshi M, et al. Role of nanotechnology in drug delivery. Int. J. Nanotechnol. Nanosci. 2017; 5: 1–29.
Yang T, Lan Y, Cao M, et al. Glycyrrhetinic acid-conjugated polymeric prodrug micelles co-delivered with doxorubicin as combination therapy treatment for liver cancer. Colloids Surf. B. 2019. doi:10.1016/j.colsurfb.2018.11.082
Barenholz YC. Doxil®—the first FDA-approved nano-drug: Lessons learned. J. Control. Release 2012; 160(2): 117–134. doi:10.1016/j.jconrel.2012.03.020
Wang B, Qiao W, Wang Y, et al. Cancer therapy based on nanomaterials and nanocarrier systems. J. Nanomater. 2010; 2010. doi:10.1155/2010/796303
Masood F. Polymeric nanoparticles for targeted drug delivery system for cancer therapy. Mater. Sci. Eng. C. 2016; 60: 569–578. doi:10.1016/j.msec.2015.11.067
Ye B, Zheng R, Ruan X, et al. Chitosan-coated doxorubicin nano-particles drug delivery system inhibits cell growth of liver cancer via p53/PRC1 pathway. Biochem. Biophys. Res. Commun. 2018; 495(1): 414–420. doi:10.1016/j.bbrc.2017.10.156
Loutfy SA, El-Din HMA, Elberry MH, et al. Synthesis, characterization and cytotoxic evaluation of chitosan nanoparticles: In vitro liver cancer model. Adv. Nat. Sci. 2016; 7(3): 035008.
Huang W, Wang W, Wang P, et al. Glycyrrhetinic acid-modified poly(ethylene glycol)-b-poly(γ-benzyl l-glutamate) micelles for liver targeting therapy. Acta Biomater. 2010. doi:10.1016/j.actbio.2010.04.021
Hanafy NAN, Quarta A, Ferraro MM, et al. Polymeric nano-micelles as novel cargo-carriers for LY2157299 liver cancer cells delivery. Int. J. Mol. Sci. 2018. doi:10.3390/ijms19030748
Immordino ML, Dosio F, Cattel L. Stealth liposomes: Review of the basic science, rationale, and clinical applications, existing and potential. Int. J. Nanomed. 2006; 1(3): 297–315.
Wang J, Wu Z, Pan G, et al. Enhanced doxorubicin delivery to hepatocellular carcinoma cells via CD147 antibody-conjugated immunoliposomes. Nanomed. 2018; 14(6): 1949–1961. doi:10.1016/j.nano.2017.09.012
Persico M, Barbarisi M, Armenia E, et al. Chitosan-coated liposomes loaded with butyric acid demonstrate anticancer and anti-inflammatory activity in human hepatoma HepG2 cells. Oncol. Rep. 2018. doi:10.3892/or.2018.6932
Noriega-Luna B, Godínez LA, Rodríguez FJ, et al. Applications of dendrimers in drug delivery agents, diagnosis, therapy, and detection. J. Nanomater. 2014; 2014: 1–19. doi:10.1155/2014/507273
Peer D, Karp JM, Hong S, et al. Nanocarriers as an emerging platform for cancer therapy. Nat. Nanotechnol. 2007; 2(12): 751–760. doi:10.1038/nnano.2007.387
Wojnarowicz J, Jesionowski T, Grześkowiak BF, et al. Dendrimer based theranostic nanostructures for combined chemo- and photothermal therapy of liver cancer cells in vitro. Colloids Surf. B. 2018; 173: 698–708. doi:10.1016/j.colsurfb.2018.10.045
Fu F, Wu Y, Zhu J, et al. Multifunctional lactobionic acid-modified dendrimers for targeted drug delivery to liver cancer cells: Investigating the role played by PEG spacer. ACS Appl. Mater. Interfaces. 2014; 6(18): 16416–16425. doi:10.1021/am504849x
Jain NK, Mody N, Tekade RK, et al. Glycyrrhizin conjugated dendrimer and multi-walled carbon nanotubes for liver specific delivery of doxorubicin. J. Nanosci. Nanotechnol. 2014; 15(2): 1088–1100. doi:10.1166/jnn.2015.9039
Bondì ML, Botto C, Amore E, et al. Lipid nanocarriers containing sorafenib inhibit colonies formation in human hepatocarcinoma cells. Int. J. Pharm. 2015; 493(1–2): 75–85. doi:10.1016/j.ijpharm.2015.07.055
Zhao X, Chen Q, Li Y, et al. Doxorubicin and curcumin co-delivery by lipid nanoparticles for enhanced treatment of diethylnitrosamine-induced hepatocellular carcinoma in mice. Eur. J. Pharm. Biopharm. 2015; 93: 27–36. doi:10.1016/j.ejpb.2015.03.003
Ebara M, Lee HJ, Aoyagi T, et al. Simultaneous drug and gene delivery from the biodegradable Poly(-caprolactone) nanofibers for the treatment of liver cancer. J. Nanosci. Nanotechnol. 2015. doi:10.1166/jnn.2015.11233
Ji Y, Xiao Y, Xu L, et al. Drug-bearing supramolecular MMP inhibitor nanofibers for inhibition of metastasis and growth of liver cancer. Adv. Sci. 2018; 5: 1700867. doi:10.1002/advs.201700867
Yang C, Yang C, Xu H, et al. Novel tumour-targeting, self-assembling peptide nanofiber as a carrier for effective curcumin delivery. Int. J. Nanomed. 2013; 197. doi:10.2147/IJN.S55875
Zhang X, Deng F, Tian J, et al. Improving the drug delivery characteristics of graphene oxide based polymer nanocomposites through the “one-pot” synthetic approach of single-electron transfer living radical polymerization. Appl. Surf. Sci. 2016; 378: 22–29. doi:10.1016/j.apsusc.2016.03.207
Shim G, Kim MG, Park JY, et al. Graphene-based nanosheets for delivery of chemotherapeutics and biological drugs. Adv. Drug Deliv. Rev. 2016; 105: 205–227. doi:10.1016/j.addr.2016.04.004
Yang H, Li H, Zhu L, et al. Lactobionic acid and carboxymethyl chitosan functionalized graphene oxide nanocomposites as targeted anticancer drug delivery systems. Carbohydr. Polym. 2016; 151: 812–820. doi:10.1016/j.carbpol.2016.06.024
Yuan Y, Zhang Y, Liu B, et al. The effects of multifunctional MiR-122-loaded graphene-gold composites on drug-resistant liver cancer. J. Nanobiotechnol. 2015; 13(1). doi:10.1186/s12951-015-0070-z
Danhier F, Ansorena E, Silva JM, et al. PLGA-based nanoparticles: An overview of biomedical applications. J. Control. Release 2012; 161(2): 505–522. doi:10.1016/j.jconrel.2012.01.043
Gao DY, Lin TT, Sung YC, et al. CXCR4-targeted lipid-coated PLGA nanoparticles deliver sorafenib and overcome acquired drug resistance in liver cancer. Biomater. 2015; 67: 194–203. doi:10.1016/j.biomaterials.2015.07.035
Dangi R, Hurkat P, Jain A, et al. Targeting liver cancer via ASGP receptor using 5-FU-loaded surface-modified PLGA nanoparticles. J. Microencapsul. 2014; 31(5): 479–487. doi:10.3109/02652048.2013.879929
Elhissi A, Ahmed W, Hassan IU, et al. Carbon nanotubes in cancer therapy and drug delivery. J. Drug Deliv. 2012; 2012: 1–10. doi:10.1155/2012/837327
He H, Xiao D, Pham-Huy LA, et al. Carbon nanotubes used as nanocarriers in drug and biomolecule delivery. Drug Delivery Approaches Nanosyst. 2017; 163–212. doi:10.1201/9781315225371
Qi X, Rui Y, Fan Y, et al. Galactosylated chitosan-grafted multiwall carbon nanotubes for pH-dependent sustained release and hepatic tumor-targeted delivery of doxorubicin in vivo. Colloids Surf. B. 2015; 133: 314–322. doi:10.1016/j.colsurfb.2015.06.003
Ji Z, Lin G, Lu Q, et al. Targeted therapy of SMMC-7721 liver cancer in vitro and in vivo with carbon nanotubes based drug delivery system. J. Colloid Interface Sci. 2012; 365(1): 143–149. doi:10.1016/j.jcis.2011.09.013
Wang Y-XJ, Leung KC-F, Zhu X-M, et al. In vivo chemoembolization and magnetic resonance imaging of liver tumours by using iron oxide nanoshell/doxorubicin/poly(vinyl alcohol) hybrid composites. Angew. Chem. Int. Ed. 2014; 53(19): 4812–4815. doi:10.1002/anie.201402144
Ye Y. Integrin targeting for tumour optical imaging. Theragnostic. 2011; 1: 102. doi:10.7150/thno/v01p0102
Zhang D, Wu M, Zeng Y, et al. Lipid micelles packaged with semiconducting polymer dots as simultaneous MRI/photoacoustic imaging and photodynamic/photothermal dual-modal therapeutic agents for liver cancer. J. Mater. Chem. B. 2016. doi:10.1039/c5tb01827g
Liu Y, Yu D, Zhang N, et al. Gadolinium-loaded polymeric nanoparticles modified with Anti-VEGF as multifunctional MRI contrast agents for the diagnosis of liver cancer. Biomater. 2011; 32(22): 5167–5176. doi:10.1016/j.biomaterials.2011.03.077
Luo K, Liu G, He B, et al. Multifunctional gadolinium-based dendritic macromolecules as liver targeting imaging probes. Biomater. 2011; 32(10): 2575–2585. doi:10.1016/j.biomaterials.2010.12.049
Park S, Kim JW, Jeong YY, et al. Drug-loaded superparamagnetic iron oxide nanoparticles for combined cancer imaging and therapy in vivo. Angew. Chem. Int. Ed. 2008; 47(29): 5362–5365. doi:10.1002/anie.200800857
Wang YXJ. Current status of superparamagnetic iron oxide contrast agents for liver magnetic resonance imaging. World J. Gastroenterol. 2015; 21(47): 13400–13402. doi:10.3748/wjg.v21.i47.13400
Arias JL, Reddy LH, Othman M, et al. Squalene based nanocomposites: a new platform for the design of multifunctional pharmaceutical theragnostic. ACS Nano. 2011; 5(2): 1513–1521. doi:10.1021/nn1034197
Olerile LD, Liu Y, Zhang B, et al. Near-infrared mediated quantum dots and paclitaxel co-loaded nanostructured lipid carriers for cancer theragnostic. Colloids Surf. B. 2017. doi:10.1016/j.colsurfb.2016.11.032
Das RK, Mohapatra S. Highly luminescent, heteroatom-doped carbon quantum dots for ultrasensitive sensing of glucosamine and targeted imaging of liver cancer cells. J. Mater. Chem. B. 2017. doi:10.1039/c6tb03141b.
Shao D, Li J, Pan Y, et al. Noninvasive theranostic imaging of HSV-TK/GCV suicide gene therapy in liver cancer by folate-targeted quantum dot-based liposomes. Biomater. Sci. 2015; 3(6): 833–841. doi:10.1039/C5BM00077G
Al-Jamal WT, Al-Jamal KT, Cakebread A, et al. Blood circulation and tissue biodistribution of lipid-quantum dot (L-QD) hybrid vesicles intravenously administered in mice. Bioconjug. Chem. 2009; 20: 1696–1702. doi:10.1021/bc900047n
Maeda H, Nakamura H, Fang J. The EPR effect for macromolecular drug delivery to solid tumours: improvement of tumor uptake, lowering of systemic toxicity, and distinct tumour imaging in vivo. Adv Drug Deliv. Rev. 2013; 65(1): 71–79. doi:10.1016/j.addr.2012.10.002
Mohamed NK, Hamad MA, Hafez MZE, et al. Nanomedicine in management of hepatocellular carcinoma: challenges and opportunities. Int. J. Cancer. 2017; 140(7): 1475–1484. doi:10.1002/ijc.30517.
Bae YH, Park K. Targeted drug delivery to tumours: Myths, reality and possibility. J. Control. Release 2011;153(3):198–205. doi:10.1016/j.jconrel.2011.06.001
Xiao K, Li Y, Luo J, et al. The effect of surface charge on in vivo biodistribution of PEG-oligocholic acid based micellar nanoparticles. Biomater. 2011; 32(13): 3435–3446. doi:10.1016/j.biomaterials.2011.01.021
Prabhu RH, Patravale VB, Joshi MD. Polymeric nanoparticles for targeted treatment in oncology: current insights. Int. J. Nanomedicine. 2015; 10: 1001–1018. doi:10.2147/IJN. S56932
Ferreira DDS, Lopes SCDA, Franco MS, et al. PH sensitive liposomes for drug delivery in cancer treatment. Ther. Deliv. 2013; 4(9): 1099–1123. doi:10.4155/tde.13.80
Xia Q, Li L, Zhao L. Silica nanoparticle-based dual-responsive nanoprodrug system for liver cancer therapy. Exp. Ther. Med. 2017; 14: 2071–2077. doi:10.3892/etm.2017.4768
Narayan R. Encyclopedia of Biomedical Engineering. Elsevier; 2018.
Walkey CD, Chan WCW. Understanding and controlling the interaction of nanomaterials with proteins in a physiological environment. Chem. Soc. Rev. 2012; 41(7): 2780–2799. doi:10.1039/c1cs15233e
Nkansah P, Antipas A, Lu Y, et al. Development and evaluation of novel solid nanodispersion system for oral delivery of poorly water-soluble drugs. J. Control. Release 2013; 169(1–2): 150–161. doi:10.1016/j.jconrel.2013.03.032
Zhou F, Shang W, Yu X, et al. Glypican-3: A promising biomarker for hepatocellular carcinoma diagnosis and treatment. Med. Res. Rev. 2018; 38(2): 741–767. doi:10.1002/med.21455
Voutila J, Reebye V, Roberts TC, et al. Development and mechanism of small activating RNA targeting CEBPA, a novel therapeutic in clinical trials for liver cancer. Mol. Ther. 2017; 25(12): 2705–2714. doi:10.1016/j.ymthe.2017.07.018
Kim Y, Jo M, Schmidt J, et al. Enhanced potency of GalNAc-conjugated antisense oligonucleotides in hepatocellular cancer models. Mol. Ther. 2019; 27(9): 1547–1557. doi:10.1016/j.ymthe.2019.06.009
Harada T, Matsumoto S, Hirota S, et al. Chemically modified antisense oligonucleotide against ARL4C inhibits primary and metastatic liver tumour growth. Mol. Cancer Ther. 2019; 18(3):602–612. doi:10.1158/1535-7163.MCT-18-0824
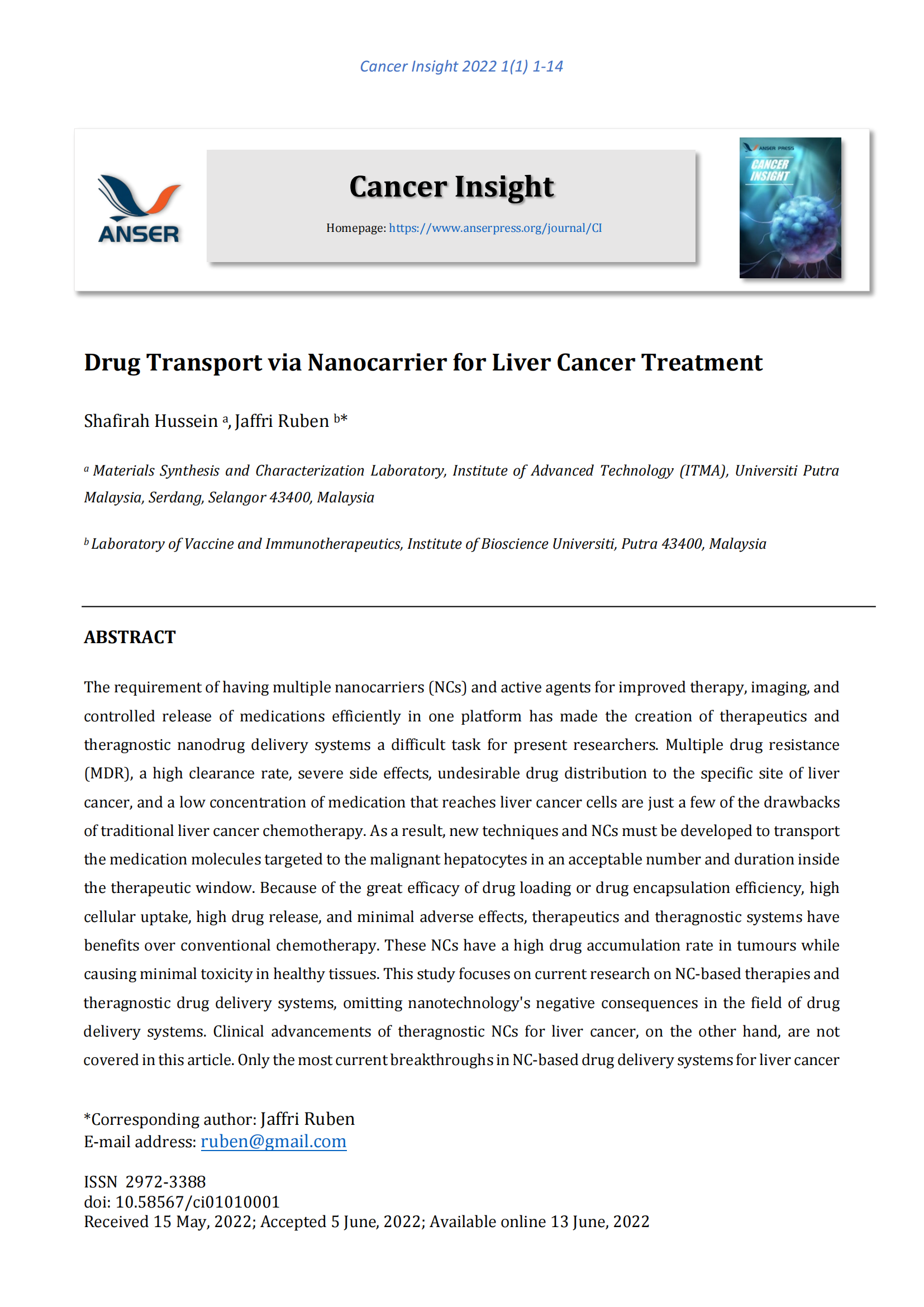